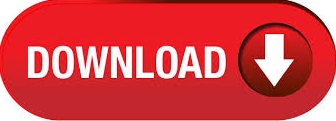

Prominent candidates for such particles are axions and axion-like particles (ALPs), and they often arise as Nambu-Goldstone bosons associated with the breakdown of global symmetries. Many of the above-mentioned extensions of the Standard Model predict not only WIMPs, but also WISPs (very weakly interacting subelectronvolt particles). Moreover, the vacuum energy density of the universe, as inferred from cosmological observations, points to the subelectronvolt range, ρ Λ ∼ meV 4.
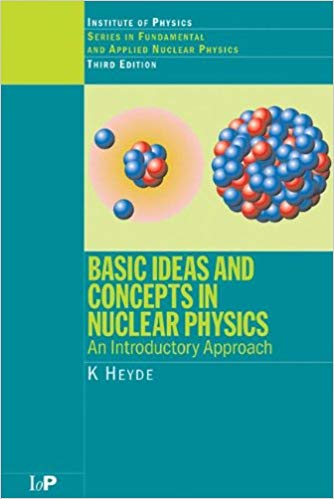

Indeed, atmospheric, reactor, and solar neutrino data strongly support the hypothesis that neutrinos have masses in the subelectronvolt range. However, there is also evidence of fundamental physics at the subelectronvolt scale. Some of these particles, such as neutralinos, are natural candidates for the constituents of cold dark matter (CDM) in the form of so-called weakly interacting massive particles (WIMPs). Indeed, most proposals to embed the Standard Model of particle physics into a more general, unified framework-notably those based on string theory or its low-energy incarnations, supergravity and supersymmetry-predict new heavy ( m≳100 GeV) particles that may be searched for at teraelectronvolt colliders. There is much circumstantial evidence that the teraelectronvolt-scale physics exploited at the LHC will provide decisive insights into fundamental questions such as the origin of particle masses, the nature of dark matter in the universe, and the unification of all forces, including gravity. We are entering an exciting time in particle physics: The Large Hadron Collider (LHC) is setting a new benchmark at the high-energy frontier and, through the collision of multiteraelectronvolt protons, is probing the structure of matter and space-time at an unprecedented level.
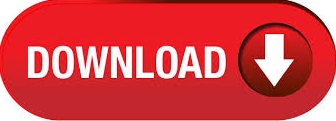